Difference between revisions of "PH Buffering in Aquifers"
m (1 revision imported) |
(Tag: Visual edit) |
||
(33 intermediate revisions by 2 users not shown) | |||
Line 1: | Line 1: | ||
Processes that inhibit pH changes in an aquifer are called pH buffering processes. These processes are important because pH is often a key control on the chemical and microbiological processes responsible for contaminant remediation. All aquifers have some natural buffering capacity, but in many cases the pH buffering capacity must be augmented to achieve optimum remediation performance. This is particularly true for remedial processes that generate acid such as microbial degradation of [[Chlorinated Solvents|chlorinated solvents]]. Considerations in choosing amendments for enhancing aquifer-buffering capacity are achieving the correct dose, the longevity of it in the aquifer, and any collateral effects. | Processes that inhibit pH changes in an aquifer are called pH buffering processes. These processes are important because pH is often a key control on the chemical and microbiological processes responsible for contaminant remediation. All aquifers have some natural buffering capacity, but in many cases the pH buffering capacity must be augmented to achieve optimum remediation performance. This is particularly true for remedial processes that generate acid such as microbial degradation of [[Chlorinated Solvents|chlorinated solvents]]. Considerations in choosing amendments for enhancing aquifer-buffering capacity are achieving the correct dose, the longevity of it in the aquifer, and any collateral effects. | ||
<div style="float:right;margin:0 0 2em 2em;">__TOC__</div> | <div style="float:right;margin:0 0 2em 2em;">__TOC__</div> | ||
+ | |||
+ | |||
'''Related Article(s):''' | '''Related Article(s):''' | ||
− | |||
− | |||
− | |||
− | ''' | + | *[[Biodegradation - Reductive Processes]] |
+ | *[[Bioremediation - Anaerobic]] | ||
+ | *[[Injection Techniques for Liquid Amendments]] | ||
+ | *[[Low pH Inhibition of Reductive Dechlorination]] | ||
+ | *[[Design Tool - Base Addition for ERD]] | ||
+ | |||
+ | |||
+ | '''Contributor(s):''' [[Dr. Miles Denham]] | ||
'''Key Resource(s):''' | '''Key Resource(s):''' | ||
− | *[http://dx.doi.org/10.1016/j.scitotenv.2009.03.029 pH Control for Enhanced Reductive Bioremediation of Chlorinated Solvent Source Zones]<ref name= "Robinson2009">Robinson, C., Barry, D.A., McCarty, P.L., Gerhard, J.I., Kouznetsova, I., 2009. pH control for enhanced reductive bioremediation of chlorinated solvent source zones. Science of the Total Environment, 407(16), 4560-4573. [http://dx.doi.org/10.1016/j.scitotenv.2009.03.029 doi:10.1016/j.scitotenv.2009.03.029]</ref> | + | |
+ | *[http://dx.doi.org/10.1016/j.scitotenv.2009.03.029 pH Control for Enhanced Reductive Bioremediation of Chlorinated Solvent Source Zones]<ref name="Robinson2009">Robinson, C., Barry, D.A., McCarty, P.L., Gerhard, J.I., Kouznetsova, I., 2009. pH control for enhanced reductive bioremediation of chlorinated solvent source zones. Science of the Total Environment, 407(16), 4560-4573. [http://dx.doi.org/10.1016/j.scitotenv.2009.03.029 doi:10.1016/j.scitotenv.2009.03.029]</ref> | ||
==Introduction== | ==Introduction== | ||
− | Buffering of pH – the resistance to pH change – in groundwater systems can be an important control on contaminant attenuation or degradation processes. This happens when aquifer buffering capacity is sufficient to counteract pH changes resulting from contaminants entering the aquifer and/or in situ remediation processes. Buffering capacity can occur naturally or be engineered by [[Injection | + | Buffering of pH – the resistance to pH change – in groundwater systems can be an important control on contaminant attenuation or degradation processes. This happens when aquifer buffering capacity is sufficient to counteract pH changes resulting from contaminants entering the aquifer and/or in situ remediation processes. Buffering capacity can occur naturally or be engineered by [[Injection Techniques for Liquid Amendments |addition of amendments]] to the groundwater system. |
==Natural Buffering Capacity== | ==Natural Buffering Capacity== | ||
Line 21: | Line 28: | ||
These reactions are reversible so that (a) an influx of acid will cause the [[wikipedia: Bicarbonate | bicarbonate]] (HCO<sub>3</sub><sup>-</sup>) and [[wikipedia: Carbonate | carbonate]] (CO<sub>3</sub><sup>-2</sup>) ions to [[wikipedia: Protonation | protonate]], consuming the acid, or (b) an influx of base will cause dissociation (deprotonation) of dissolved carbonic acid (H<sub>2</sub>CO<sub>3</sub>) and the bicarbonate ion to consume the base. Maximum resistance to pH change (buffering capacity) occurs when pH equals the dissociation constants of carbonic acid (pH = 6.3 @ 25°C) or bicarbonate ion (pH = 10.3 @ 25°C). Figure 1 illustrates the dissolved carbonate system. | These reactions are reversible so that (a) an influx of acid will cause the [[wikipedia: Bicarbonate | bicarbonate]] (HCO<sub>3</sub><sup>-</sup>) and [[wikipedia: Carbonate | carbonate]] (CO<sub>3</sub><sup>-2</sup>) ions to [[wikipedia: Protonation | protonate]], consuming the acid, or (b) an influx of base will cause dissociation (deprotonation) of dissolved carbonic acid (H<sub>2</sub>CO<sub>3</sub>) and the bicarbonate ion to consume the base. Maximum resistance to pH change (buffering capacity) occurs when pH equals the dissociation constants of carbonic acid (pH = 6.3 @ 25°C) or bicarbonate ion (pH = 10.3 @ 25°C). Figure 1 illustrates the dissolved carbonate system. | ||
[[File:Denham pH buffering Fig1.jpg|thumbnail|550px|left|Figure 1. Graphic showing the dissolved carbonate system.]] | [[File:Denham pH buffering Fig1.jpg|thumbnail|550px|left|Figure 1. Graphic showing the dissolved carbonate system.]] | ||
− | For a system open to a large reservoir of carbon dioxide, the buffering capacity (assuming no soluble minerals are present) is a function of the partial pressure of carbon dioxide in the reservoir. In a closed system, the buffering capacity is a function of the total dissolved carbonate (H<sub>2</sub>CO<sub>3</sub>, HCO<sub>3</sub><sup>-</sup>, and CO<sub>3</sub><sup>-2</sup>). The buffering capacity of groundwater is measured with an alkalinity titration<ref>U.S. Geological Survey, 2015. National field manual for the collection of water-quality field data, Alkalinity and acid neutralizing capacity. US Geological Survey Techniques of Water-Resources Investigations, Book 9, Chap. A6., Section 6.6. [ | + | For a system open to a large reservoir of carbon dioxide, the buffering capacity (assuming no soluble minerals are present) is a function of the partial pressure of carbon dioxide in the reservoir. In a closed system, the buffering capacity is a function of the total dissolved carbonate (H<sub>2</sub>CO<sub>3</sub>, HCO<sub>3</sub><sup>-</sup>, and CO<sub>3</sub><sup>-2</sup>). The buffering capacity of groundwater is measured with an alkalinity titration<ref>U.S. Geological Survey, 2015. National field manual for the collection of water-quality field data, Alkalinity and acid neutralizing capacity. US Geological Survey Techniques of Water-Resources Investigations, Book 9, Chap. A6., Section 6.6. [//www.enviro.wiki/images/f/ff/USGS-2015-Natl_Field_Manual.pdf Report pdf]</ref><ref>American Public Health Association, American Water Works Association and Water Environment Federation. Standard Method 2320 Alkalinity, Standard methods for the examination of water and wastewater.</ref>. Commercial field kits are available for measuring alkalinity. In most aquifers at constant total dissolved carbonate, the bicarbonate ion will dominate alkalinity, with the carbonate ion becoming more prominent as pH exceeds 10. Nevertheless, other ions contribute to alkalinity including hydroxyl, phosphate, silicate, and borate, but only dominate in rare cases. The units of alkalinity are often reported as mg CaCO<sub>3</sub>/L (milligrams of CaCO<sub>3</sub> per liter): |
− | Alkalinity (mg CaCO<sub>3</sub>/L) = Alkalinity (milliequivalents/L) x | + | Alkalinity (mg CaCO<sub>3</sub>/L) = Alkalinity (milliequivalents/L) x 50 (mg CaCO<sub>3</sub>/milliequivalent) |
===Minerals=== | ===Minerals=== | ||
Line 32: | Line 39: | ||
===Mineral Surfaces=== | ===Mineral Surfaces=== | ||
Surfaces of clay minerals and similarly-sized oxides in aquifers can also act as pH buffers. The surfaces of these minerals can reversibly bind and release hydrogen ions as pH changes (Fig. 2). | Surfaces of clay minerals and similarly-sized oxides in aquifers can also act as pH buffers. The surfaces of these minerals can reversibly bind and release hydrogen ions as pH changes (Fig. 2). | ||
− | [[File:Denham pH buffering Fig2.jpg|thumbnail|550 px|right|Figure 2. Diagram of mineral surface exchanging hydrogen ions with varying pH<ref>ITRC, 2010. A Decision Framework for Applying Monitored Natural Attenuation Processes to Metals and Radionuclides, Interstate Technology and Regulatory Council, Technical/Regulatory Guidance AMPR-1. [ | + | [[File:Denham pH buffering Fig2.jpg|thumbnail|550 px|right|Figure 2. Diagram of mineral surface exchanging hydrogen ions with varying pH<ref>ITRC, 2010. A Decision Framework for Applying Monitored Natural Attenuation Processes to Metals and Radionuclides, Interstate Technology and Regulatory Council, Technical/Regulatory Guidance AMPR-1. [//www.enviro.wiki/images/a/ac/ITRC-2010-A_Decision_Framework.pdf Report pdf]</ref>.]] |
− | Broken bonds at mineral surfaces leave oxygens that are not fully bonded and act as weak acid anions that adsorb hydrogen ions (and other cations). Influxing acid can be neutralized by adsorbing hydrogen ions on negatively charged surface sites and influxing base can be neutralized by adsorbing to positively charged sites. The zero point of charge is the pH value at which the net surface charge density is zero. The buffering capacity for acid or base depends on how far the pH of the system is above or below the zero point of charge. The point of zero charge is different for different minerals and lists are available from many sources<ref name="Langmuir1997"/>. | + | Broken bonds at mineral surfaces leave oxygens that are not fully bonded and act as weak acid anions that adsorb hydrogen ions (and other cations). Influxing acid can be neutralized by adsorbing hydrogen ions on negatively charged surface sites and influxing base can be neutralized by adsorbing to positively charged sites. The zero point of charge is the pH value at which the net surface charge density is zero. The buffering capacity for acid or base depends on how far the pH of the system is above or below the zero point of charge. The point of zero charge is different for different minerals and lists are available from many sources<ref name="Langmuir1997" />. |
===Total Buffering Capacity=== | ===Total Buffering Capacity=== | ||
− | The total aquifer buffering capacity is the sum of the buffering capacity of the groundwater plus that of the aquifer minerals and is determined by acid-base titration. A variety of methods exist for measuring buffering capacity (examples<ref>Nelson, P.N., Su, N., 2010. Soil pH buffering capacity: a descriptive function and its application to some acidic tropical soils. Soil Research, 48(3), 201-207. [http://dx.doi.org/10.1071/SR09150 doi: 10.1071/SR09150]</ref><ref>Rong-liang, Q., Qing, Z.Y.N., Yun-ni, Z., 1998. Solid components and acid buffering capacity of soils in South China. Journal of Environmental Sciences, 10(2). [ | + | The total aquifer buffering capacity is the sum of the buffering capacity of the groundwater plus that of the aquifer minerals and is determined by acid-base titration. A variety of methods exist for measuring buffering capacity (examples<ref>Nelson, P.N., Su, N., 2010. Soil pH buffering capacity: a descriptive function and its application to some acidic tropical soils. Soil Research, 48(3), 201-207. [http://dx.doi.org/10.1071/SR09150 doi: 10.1071/SR09150]</ref><ref>Rong-liang, Q., Qing, Z.Y.N., Yun-ni, Z., 1998. Solid components and acid buffering capacity of soils in South China. Journal of Environmental Sciences, 10(2). [//www.enviro.wiki/images/5/52/Rong-liang-1998-Solid_components.pdf Report pdf]</ref>, or Standard Method 2320<ref>American Public Health Association, American Water Works Association and Water Environment Federation, 1998. Standard methods for the examination of water and wastewater.</ref> can be modified for solids), but the method should be consistent with the end-use of the measurement. The slow kinetics of some pH buffering reactions means that various methods may yield different results depending upon equilibration time. When the end-use is environmental remediation, relatively short equilibration times (several days to two weeks), that don’t capture the buffering capacity of silicate dissolution are most appropriate for common aquifer systems. |
==Engineered pH Buffering== | ==Engineered pH Buffering== | ||
There are many cases where raising and buffering pH are beneficial to the environmental remediation process. Anoxic limestone drains have been used to raise the pH of acid mine drainage by reacting the acidic metal contaminated water with calcite, promoting precipitation of the contaminant metals. Likewise, groundwater contaminated by metals and radionuclides is often acidic and raising pH to natural values can increase in situ adsorption and precipitation of the contaminants<ref>Bethke, C., Bethke, C. M., 2004. In situ treatment process to remove metal contamination from groundwater. U.S. Patent Application 10/777,390.</ref>. Raising and buffering pH can also be necessary during remediation of organic compounds. | There are many cases where raising and buffering pH are beneficial to the environmental remediation process. Anoxic limestone drains have been used to raise the pH of acid mine drainage by reacting the acidic metal contaminated water with calcite, promoting precipitation of the contaminant metals. Likewise, groundwater contaminated by metals and radionuclides is often acidic and raising pH to natural values can increase in situ adsorption and precipitation of the contaminants<ref>Bethke, C., Bethke, C. M., 2004. In situ treatment process to remove metal contamination from groundwater. U.S. Patent Application 10/777,390.</ref>. Raising and buffering pH can also be necessary during remediation of organic compounds. | ||
− | [[File:Denham pH buffering | + | [[File:Denham pH buffering Fig3c.PNG|thumbnail|350 px|right|Figure 3. Titration of two acids (0.5 mM HCl and 1.0 mM acetic acid with 10 mM NaOH).]] |
To calculate the amount of base or buffer required to increase aquifer pH, the groundwater acidity should first be measured. ''Acidity should not be confused with pH''. Acidity is the amount of base required to neutralize acids present in a water sample. Since acids can disassociate to different extents, two solutions with the same pH can have different acidities. Figure 3 shows how the pH of solutions containing 0.5 N HCl and 1.0 N acetic acid change as NaOH is added. The initial pH of the acetic acid solution is higher than that of the HCl solution, yet the acetic acid solution has a higher acidity. | To calculate the amount of base or buffer required to increase aquifer pH, the groundwater acidity should first be measured. ''Acidity should not be confused with pH''. Acidity is the amount of base required to neutralize acids present in a water sample. Since acids can disassociate to different extents, two solutions with the same pH can have different acidities. Figure 3 shows how the pH of solutions containing 0.5 N HCl and 1.0 N acetic acid change as NaOH is added. The initial pH of the acetic acid solution is higher than that of the HCl solution, yet the acetic acid solution has a higher acidity. | ||
Line 49: | Line 56: | ||
In some aquifers, the pH is low due to high concentrations of dissolved CO<sub>2</sub>. Below the water table, the high pressure prevents degassing, however, when a water sample is brought to the surface, the reduced pressure results in CO<sub>2</sub> degassing. If this occurs, pH will increase and the measured acidity will not be representative of the "in situ" conditions at depth. | In some aquifers, the pH is low due to high concentrations of dissolved CO<sub>2</sub>. Below the water table, the high pressure prevents degassing, however, when a water sample is brought to the surface, the reduced pressure results in CO<sub>2</sub> degassing. If this occurs, pH will increase and the measured acidity will not be representative of the "in situ" conditions at depth. | ||
− | Acid is also produced during remediation of organic solvents during anaerobic dechlorination<ref name= "Robinson2009"/> provides a good discussion of mechanisms) and pH buffering is often required to sustain the process<ref>ITRC, 1998. Technical and Regulatory Requirements for Enhanced In Situ Bioremediation of Chlorinated Solvents in Groundwater, Interstate Technology and Regulatory Cooperation. [ | + | Acid is also produced during remediation of organic solvents during anaerobic dechlorination (<ref name="Robinson2009" /> provides a good discussion of mechanisms) and pH buffering is often required to sustain the process<ref>ITRC, 1998. Technical and Regulatory Requirements for Enhanced In Situ Bioremediation of Chlorinated Solvents in Groundwater, Interstate Technology and Regulatory Cooperation. [//www.enviro.wiki/images/1/1a/ITRC-1998-Tech_and_Reg_Req.pdf Report pdf]</ref><ref>Air Force Center for Engineering and the Environment (AFCEE), 2004. Principles and Practices of Enhanced Anaerobic Bioremediation of Chlorinated Solvents. Parsons Corporation. Air Force Center for Environmental Excellence, Naval Facilities Engineering Service Center, and Environmental Security Technology Certification Program. [//www.enviro.wiki/images/d/d5/AFCEE_Principles_and_Practices.pdf Report pdf]</ref><ref>Henry, B., 2010. Loading Rates and Impacts of Substrate Delivery for Enhanced Anaerobic Bioremediation, ESTCP Project ER-0627. [https://www.serdp-estcp.org/content/download/10597/130781/file/ER-200627 Report pdf]</ref>. In the remediation literature, the most commonly mentioned buffering agents are forms of bicarbonate and carbonate<ref name="Robinson2009" /><ref>Delgado, A.G., Parameswaran, P., Fajardo-Williams, D., Halden, R.U., Krajmalnik-Brown, R., 2012. Role of bicarbonate as a pH buffer and electron sink in microbial dechlorination of chloroethenes. Microbial Cell Factories, 11(1), 128. [http://microbialcellfactories.biomedcentral.com/articles/10.1186/1475-2859-11-128 doi: 10.1186/1475-2859-11-128]</ref>. Other reagents used to raise and buffer pH include phosphate<ref>Major, D., Aziz, C., Watling, M., Gossett, J., Spain, J., Nishino, S., 2010. Enhancing Natural Attenuation through Bioaugmentation with Aerobic Bacteria that Degrade cis-1, 2-Dichloroethene. ESTCP Project ER-200516. [https://www.serdp-estcp.org/Program-Areas/Environmental-Restoration/Contaminated-Groundwater/ER-200516 www.serdp-estcp.org/Program-Areas/Environmental-Restoration/Contaminated-Groundwater/ER-200516 ER-200516]</ref>, magnesium hydroxide<ref>ESTCP, 2006. Protocol for enhanced in situ bioremediation using emulsified edible oil. Solutions-IES, Inc., Raleigh, NC. [https://www.serdp-estcp.org/Tools-and-Training/Environmental-Restoration/Groundwater-Plume-Treatment/Protocol-for-Enhanced-In-Situ-Bioremediation-Using-Emulsified-Edible-Oil ESTCP Protocol Webpage]</ref>, and silicate minerals<ref>Lacroix, E., Brovelli, A., Barry, D.A., Holliger, C., 2014. Use of silicate minerals for pH control during reductive dechlorination of chloroethenes in batch cultures of different microbial consortia. Applied and Environmental Microbiology, 80(13), 3858-3867. [http://dx.doi.org/10.1128/aem.00493-14 doi:10.1128/AEM.00493-14]</ref>. |
==Bases== | ==Bases== | ||
Line 59: | Line 66: | ||
==Estimating Amendment Dosage== | ==Estimating Amendment Dosage== | ||
− | When manipulating and buffering pH during remediation, it is important to know the dosage of amendment required to achieve the desired results. The goal is to keep groundwater pH within the optimal range. Dose amount is based on an estimate of mass of acid produced during the remediation process and the acid buffering capacity of the aquifer solids. BUCHLORAC<ref name= "Robinson2009"/> is a geochemical model that incorporates thermodynamics and kinetics of processes occurring during anaerobic dechlorination of solvents that can be used to estimate possible amendment dosage results. | + | When manipulating and buffering pH during remediation, it is important to know the dosage of amendment required to achieve the desired results. The goal is to keep groundwater pH within the optimal range. Dose amount is based on an estimate of mass of acid produced during the remediation process and the acid buffering capacity of the aquifer solids. BUCHLORAC<ref name="Robinson2009" /> is a geochemical model that incorporates thermodynamics and kinetics of processes occurring during anaerobic dechlorination of solvents that can be used to estimate possible amendment dosage results. |
==References== | ==References== |
Latest revision as of 21:44, 26 April 2022
Processes that inhibit pH changes in an aquifer are called pH buffering processes. These processes are important because pH is often a key control on the chemical and microbiological processes responsible for contaminant remediation. All aquifers have some natural buffering capacity, but in many cases the pH buffering capacity must be augmented to achieve optimum remediation performance. This is particularly true for remedial processes that generate acid such as microbial degradation of chlorinated solvents. Considerations in choosing amendments for enhancing aquifer-buffering capacity are achieving the correct dose, the longevity of it in the aquifer, and any collateral effects.
Related Article(s):
- Biodegradation - Reductive Processes
- Bioremediation - Anaerobic
- Injection Techniques for Liquid Amendments
- Low pH Inhibition of Reductive Dechlorination
- Design Tool - Base Addition for ERD
Contributor(s): Dr. Miles Denham
Key Resource(s):
Introduction
Buffering of pH – the resistance to pH change – in groundwater systems can be an important control on contaminant attenuation or degradation processes. This happens when aquifer buffering capacity is sufficient to counteract pH changes resulting from contaminants entering the aquifer and/or in situ remediation processes. Buffering capacity can occur naturally or be engineered by addition of amendments to the groundwater system.
Natural Buffering Capacity
In natural aqueous systems, pH buffers are predominantly weak acid anions that easily bind and release hydrogen ions. The most common are the weak acid anions produced by dissolved carbon dioxide (CO2)[2][3]. When dissolved in water, carbon dioxide combines with water as carbonic acid (H2CO3). Carbonic acid then undergoes a series of reactions, creating a buffer system:
These reactions are reversible so that (a) an influx of acid will cause the bicarbonate (HCO3-) and carbonate (CO3-2) ions to protonate, consuming the acid, or (b) an influx of base will cause dissociation (deprotonation) of dissolved carbonic acid (H2CO3) and the bicarbonate ion to consume the base. Maximum resistance to pH change (buffering capacity) occurs when pH equals the dissociation constants of carbonic acid (pH = 6.3 @ 25°C) or bicarbonate ion (pH = 10.3 @ 25°C). Figure 1 illustrates the dissolved carbonate system.
For a system open to a large reservoir of carbon dioxide, the buffering capacity (assuming no soluble minerals are present) is a function of the partial pressure of carbon dioxide in the reservoir. In a closed system, the buffering capacity is a function of the total dissolved carbonate (H2CO3, HCO3-, and CO3-2). The buffering capacity of groundwater is measured with an alkalinity titration[4][5]. Commercial field kits are available for measuring alkalinity. In most aquifers at constant total dissolved carbonate, the bicarbonate ion will dominate alkalinity, with the carbonate ion becoming more prominent as pH exceeds 10. Nevertheless, other ions contribute to alkalinity including hydroxyl, phosphate, silicate, and borate, but only dominate in rare cases. The units of alkalinity are often reported as mg CaCO3/L (milligrams of CaCO3 per liter):
Alkalinity (mg CaCO3/L) = Alkalinity (milliequivalents/L) x 50 (mg CaCO3/milliequivalent)
Minerals
The buffering capacity of an aquifer is not made up solely of the groundwater alkalinity. Minerals can contribute substantial buffering capacity, particularly in aquifers that contain calcium carbonate (the mineral calcite; CaCO3). When acid enters an aquifer containing calcite, the calcite dissolves releasing carbonate ions. If the pH is <10.3, carbonate ions will combine with hydrogen ions according to the reactions listed above, thereby buffering the pH against the acid influx. Conversely, influx of alkaline water can cause calcite to precipitate, removing carbonate ions from solution. In response, some bicarbonate ions will deprotonate, buffering the pH against the increase. Aquifers in limestones or other rocks rich in calcite have higher buffering capacities and groundwater alkalinities than aquifers containing no calcite.
Other minerals common to aquifers can also act like pH buffers. Silicate minerals such as feldspars and clays will dissolve by consuming acid in acidic conditions. Under high pH conditions, they dissolve by consuming base. These minerals dissolve incongruently and their reactions are not reversible. Therefore, they are not true pH buffers, but they do provide resistance to pH change in groundwater. However, silicate mineral reactions are generally slow compared to carbonate minerals and dissolution of most native silicate minerals will not be sufficient to counteract pH change caused by the influx of an acidic contamination plume or vigorous microbial processes that produce acid.
Mineral Surfaces
Surfaces of clay minerals and similarly-sized oxides in aquifers can also act as pH buffers. The surfaces of these minerals can reversibly bind and release hydrogen ions as pH changes (Fig. 2).
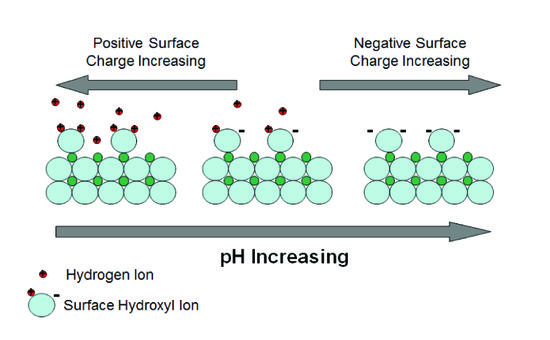
Broken bonds at mineral surfaces leave oxygens that are not fully bonded and act as weak acid anions that adsorb hydrogen ions (and other cations). Influxing acid can be neutralized by adsorbing hydrogen ions on negatively charged surface sites and influxing base can be neutralized by adsorbing to positively charged sites. The zero point of charge is the pH value at which the net surface charge density is zero. The buffering capacity for acid or base depends on how far the pH of the system is above or below the zero point of charge. The point of zero charge is different for different minerals and lists are available from many sources[2].
Total Buffering Capacity
The total aquifer buffering capacity is the sum of the buffering capacity of the groundwater plus that of the aquifer minerals and is determined by acid-base titration. A variety of methods exist for measuring buffering capacity (examples[7][8], or Standard Method 2320[9] can be modified for solids), but the method should be consistent with the end-use of the measurement. The slow kinetics of some pH buffering reactions means that various methods may yield different results depending upon equilibration time. When the end-use is environmental remediation, relatively short equilibration times (several days to two weeks), that don’t capture the buffering capacity of silicate dissolution are most appropriate for common aquifer systems.
Engineered pH Buffering
There are many cases where raising and buffering pH are beneficial to the environmental remediation process. Anoxic limestone drains have been used to raise the pH of acid mine drainage by reacting the acidic metal contaminated water with calcite, promoting precipitation of the contaminant metals. Likewise, groundwater contaminated by metals and radionuclides is often acidic and raising pH to natural values can increase in situ adsorption and precipitation of the contaminants[10]. Raising and buffering pH can also be necessary during remediation of organic compounds.
To calculate the amount of base or buffer required to increase aquifer pH, the groundwater acidity should first be measured. Acidity should not be confused with pH. Acidity is the amount of base required to neutralize acids present in a water sample. Since acids can disassociate to different extents, two solutions with the same pH can have different acidities. Figure 3 shows how the pH of solutions containing 0.5 N HCl and 1.0 N acetic acid change as NaOH is added. The initial pH of the acetic acid solution is higher than that of the HCl solution, yet the acetic acid solution has a higher acidity.
Acidity is measured by Standard Method 2310[11] in which a water sample is titrated to a specified end point with a strong base. Traditionally, an end point pH of 3.7 is used for strong acids (methyl orange acidity) and an end point 8.3 is used for weak acids (phenolphthalein or total acidity) such as dissolved CO2.
In some aquifers, the pH is low due to high concentrations of dissolved CO2. Below the water table, the high pressure prevents degassing, however, when a water sample is brought to the surface, the reduced pressure results in CO2 degassing. If this occurs, pH will increase and the measured acidity will not be representative of the "in situ" conditions at depth.
Acid is also produced during remediation of organic solvents during anaerobic dechlorination ([1] provides a good discussion of mechanisms) and pH buffering is often required to sustain the process[12][13][14]. In the remediation literature, the most commonly mentioned buffering agents are forms of bicarbonate and carbonate[1][15]. Other reagents used to raise and buffer pH include phosphate[16], magnesium hydroxide[17], and silicate minerals[18].
Bases
Table 1 lists common basic salts used to raise pH and allows a comparison of various physical properties. Bases are injected into the subsurface as solutions or solids to neutralize the acidity of the groundwater and mineral surfaces. Typically, the equivalents of base needed to neutralize the acidity of the system is measured or calculated to determine the amount of base, in terms of alkalinity, to be injected. However, there are other factors to consider when raising aquifer pH. Injection of high-pH solutions in unconsolidated sediments can cause clay minerals to disperse and clog the formation near the well. At pH values significantly >10, undesirable dissolution/reprecipitation of silicate minerals may clog the formation. Likewise, elevated pH values may inhibit desirable microbial growth.
Different base solutions have different pH values at the same alkalinity. For example, sodium bicarbonate (NaHCO3) solution has a pH of 8.3 at an alkalinity of 100 mg CaCO3/L, whereas sodium carbonate (Na2CO3) solution has a pH of 10.3 (Table 1). Hydroxides of sodium, potassium, and calcium all have a pH of 11 at the target alkalinity of 100 mg CaCO3/L. Milk of magnesia (Mg(OH) 2) has a limited solubility and an equilibrium solution will have an alkalinity <100 mg CaCO3/L. Nevertheless, when delivered to the subsurface as a solid it provides a long-lasting source of alkalinity to react with acid produced during processes such as anaerobic dechlorination of solvents. Hence, the choice of bases depends on site conditions and the specific purpose of raising pH in the subsurface.
Estimating Amendment Dosage
When manipulating and buffering pH during remediation, it is important to know the dosage of amendment required to achieve the desired results. The goal is to keep groundwater pH within the optimal range. Dose amount is based on an estimate of mass of acid produced during the remediation process and the acid buffering capacity of the aquifer solids. BUCHLORAC[1] is a geochemical model that incorporates thermodynamics and kinetics of processes occurring during anaerobic dechlorination of solvents that can be used to estimate possible amendment dosage results.
References
- ^ 1.0 1.1 1.2 1.3 Robinson, C., Barry, D.A., McCarty, P.L., Gerhard, J.I., Kouznetsova, I., 2009. pH control for enhanced reductive bioremediation of chlorinated solvent source zones. Science of the Total Environment, 407(16), 4560-4573. doi:10.1016/j.scitotenv.2009.03.029
- ^ 2.0 2.1 Langmuir, D., 1997. Aqueous Environmental Geochemistry. Prentice-Hall, Inc. Upper Saddle River, NJ. ISBN 0-02-367412-1
- ^ Drever, J.I., 1997. The Geochemistry of Natural Waters: Surface and Groundwater Environments. Prentice-Hall, Inc. ISBN 0132727900.
- ^ U.S. Geological Survey, 2015. National field manual for the collection of water-quality field data, Alkalinity and acid neutralizing capacity. US Geological Survey Techniques of Water-Resources Investigations, Book 9, Chap. A6., Section 6.6. Report pdf
- ^ American Public Health Association, American Water Works Association and Water Environment Federation. Standard Method 2320 Alkalinity, Standard methods for the examination of water and wastewater.
- ^ ITRC, 2010. A Decision Framework for Applying Monitored Natural Attenuation Processes to Metals and Radionuclides, Interstate Technology and Regulatory Council, Technical/Regulatory Guidance AMPR-1. Report pdf
- ^ Nelson, P.N., Su, N., 2010. Soil pH buffering capacity: a descriptive function and its application to some acidic tropical soils. Soil Research, 48(3), 201-207. doi: 10.1071/SR09150
- ^ Rong-liang, Q., Qing, Z.Y.N., Yun-ni, Z., 1998. Solid components and acid buffering capacity of soils in South China. Journal of Environmental Sciences, 10(2). Report pdf
- ^ American Public Health Association, American Water Works Association and Water Environment Federation, 1998. Standard methods for the examination of water and wastewater.
- ^ Bethke, C., Bethke, C. M., 2004. In situ treatment process to remove metal contamination from groundwater. U.S. Patent Application 10/777,390.
- ^ American Public Health Association, American Water Works Association and Water Environment Federation. Standard Method 2310 Acidity, In standard methods for the examination of water and wastewater.
- ^ ITRC, 1998. Technical and Regulatory Requirements for Enhanced In Situ Bioremediation of Chlorinated Solvents in Groundwater, Interstate Technology and Regulatory Cooperation. Report pdf
- ^ Air Force Center for Engineering and the Environment (AFCEE), 2004. Principles and Practices of Enhanced Anaerobic Bioremediation of Chlorinated Solvents. Parsons Corporation. Air Force Center for Environmental Excellence, Naval Facilities Engineering Service Center, and Environmental Security Technology Certification Program. Report pdf
- ^ Henry, B., 2010. Loading Rates and Impacts of Substrate Delivery for Enhanced Anaerobic Bioremediation, ESTCP Project ER-0627. Report pdf
- ^ Delgado, A.G., Parameswaran, P., Fajardo-Williams, D., Halden, R.U., Krajmalnik-Brown, R., 2012. Role of bicarbonate as a pH buffer and electron sink in microbial dechlorination of chloroethenes. Microbial Cell Factories, 11(1), 128. doi: 10.1186/1475-2859-11-128
- ^ Major, D., Aziz, C., Watling, M., Gossett, J., Spain, J., Nishino, S., 2010. Enhancing Natural Attenuation through Bioaugmentation with Aerobic Bacteria that Degrade cis-1, 2-Dichloroethene. ESTCP Project ER-200516. www.serdp-estcp.org/Program-Areas/Environmental-Restoration/Contaminated-Groundwater/ER-200516 ER-200516
- ^ ESTCP, 2006. Protocol for enhanced in situ bioremediation using emulsified edible oil. Solutions-IES, Inc., Raleigh, NC. ESTCP Protocol Webpage
- ^ Lacroix, E., Brovelli, A., Barry, D.A., Holliger, C., 2014. Use of silicate minerals for pH control during reductive dechlorination of chloroethenes in batch cultures of different microbial consortia. Applied and Environmental Microbiology, 80(13), 3858-3867. doi:10.1128/AEM.00493-14