Difference between revisions of "Stream Restoration"
Debra Tabron (talk | contribs) (Created page with "Stream restoration is often performed to reduce the effects of stressors on the environment and return stream structure and function to pre-disturbance conditions. Often, rest...") |
Debra Tabron (talk | contribs) |
||
Line 96: | Line 96: | ||
===Long-Term Effects of Restoration (2017-2019)=== | ===Long-Term Effects of Restoration (2017-2019)=== | ||
From May 2017 through January 2019, post-restoration measurements were made ~14 years after the CWD additions in 7 streams (3 unrestored, 4 restored) on a monthly and seasonal basis (compare Figure 4 to Figure 5). This study design enabled the long-term assessment of CWD dam additions as a restoration tool on stream ecosystem structure and function. The most immediate finding is that the CWD dams are still in place 14 y after their initial installation; however, preliminary analyses suggest no long-term benefits of CWD dams on stream ecosystem structure and function. | From May 2017 through January 2019, post-restoration measurements were made ~14 years after the CWD additions in 7 streams (3 unrestored, 4 restored) on a monthly and seasonal basis (compare Figure 4 to Figure 5). This study design enabled the long-term assessment of CWD dam additions as a restoration tool on stream ecosystem structure and function. The most immediate finding is that the CWD dams are still in place 14 y after their initial installation; however, preliminary analyses suggest no long-term benefits of CWD dams on stream ecosystem structure and function. | ||
− | + | </br> | |
+ | </br> | ||
==References== | ==References== | ||
<references /> | <references /> |
Revision as of 13:49, 8 October 2019
Stream restoration is often performed to reduce the effects of stressors on the environment and return stream structure and function to pre-disturbance conditions. Often, restoration projects aim to improve water quality and in-stream habitat, manage riparian zones, stabilize stream banks, and allow fish to pass barriers. Stream restorations are commonly carried out across the United States, although very few studies have assessed and monitored the long-term effects of restoration; thus, the outcomes of stream restorations are generally not well documented. Of the many possible stream restoration approaches, one prominent approach in low-gradient, sandy-bottomed streams is the addition of coarse woody debris (CWD) to improve in-stream habitat. In an experimental study summarized here, debris dams were added to 4 streams at Fort Benning Military Installation, Georgia, to mitigate the impacts of military training activities. Results were compared to 4 control streams that were not restored. There were some short-term (< 3 y) changes to stream ecosystem structure and function, but thus far, minimal long-term (~14 y) effects on these streams have been evident.
Contents
- 1 Stream Restoration: Definitions and Scope
- 2 Stressors to Stream Ecosystems That Motivate Restoration
- 3 Restoration Goals and Assessment of Efficacy: The Importance of Monitoring
- 4 Stream Restoration Techniques
- 5 Case Study: Assessment of In-Stream Restoration on DoD Land at Fort Benning Military Installation
- 6 References
- 7 See Also
Related Article(s):
CONTRIBUTOR(S): Dr. Natalie Griffiths, Dan Isenberg, Sam Bickley, Dr. Brian Helms, and Dr. Jack Feminella
Key Resource(s):
- Synthesizing US River Restoration Efforts[1]
- Riparian Ecosystem Management at Military Installations: Determination of Impacts and Evaluation of Restoration and Enhancement Strategies[2]
- Restoration of Aquatic Ecosystems: Science, Technology, and Public Policy[3]
- Global Review of the Physical and Biological Effectiveness of Stream Habitat Rehabilitation Techniques[4]
Stream Restoration: Definitions and Scope
Stream restoration is an overarching term used to describe the process of returning stream structure and function to pre-disturbance conditions[3]. This definition of “restoration” differs from “reclamation” and “rehabilitation” in that the latter two terms describe altering a system to meet various human needs[3]. Stream restorations often focus on improving the ecological condition within the stream itself, but it is important to recognize that streams are part of larger watersheds, with both upstream-downstream and lateral connections (i.e., to riparian zones and floodplains[3]. Therefore, small-scale, in-stream restorations may have little impact if larger-scale impacts (e.g., land disturbances) are not addressed[3][5][4][6]. Instead, watershed-scale approaches focusing on improving land management practices within the watershed and also applying multiple, complementary stream and riparian restoration approaches have been recommended[3][7][5][6]).
As an example, Roni et al. (2002)[8] presented a hierarchical approach for restoration, with this approach specifically developed for improving fish habitat in forests and rangelands. They suggested that managers prioritize protecting intact (i.e., non-degraded) stream reaches that have high-quality habitat for fishes. Subsequent efforts should then focus on reconnecting high-quality habitats that have been disconnected hydrologically, followed by restoring ecosystem processes (e.g., restoring riparian processes by livestock exclusion), and lastly focusing on improving in-stream habitat through habitat augmentation practices (e.g., large wood or boulder additions).
Stressors to Stream Ecosystems That Motivate Restoration
Headwater streams are important components of river networks and, like most waterways, they are also vulnerable to degradation from anthropogenic and natural sources[9]. Because of their close hydrologic connection to the surrounding terrestrial environment, headwater streams can be particularly influenced by land disturbances[10][11][12]. Further, the hydrologic connectivity of river networks means that disturbances in headwaters can influence downstream ecosystems[13]. Thus, minimizing human impacts in headwater streams is important not only for protecting these ecosystems and their biodiversity but also because of the multiple services they provide to downstream ecosystems (e.g., water for drinking, bathing, recreation, consumption of fish, etc.).
A survey of stream restorations in the southeastern US revealed that environmental degradation, mitigation, and public safety were the main factors motivating stream restorations[14]. Environmental stressors include changes in land use (e.g., forestland conversion) and hydrology (e.g., water withdrawals) in the surrounding watershed[15][6]. These factors, in turn, can reduce water quality, alter water quantity, degrade in-stream habitat and associated aquatic biodiversity, and alter availability and quality of food resources for biota (e.g., organic matter inputs[3]). Therefore, the five most common goals of stream restoration projects are:
- improve water quality;
- manage riparian zones;
- improve in-stream habitat;
- allow for fish passage and
- stabilize stream banks[1]
Restoration Goals and Assessment of Efficacy: The Importance of Monitoring
Identification of a restoration project’s mission, goals, and objectives is necessary in order to evaluate the ecological success of that restoration[3][7]. Central to this approach is the implementation of pre-restoration and post-restoration monitoring at appropriate spatial and temporal scales[3][7]. Despite the large number of restorations that have taken place at a combined cost of >$1 billion per year in the United States[1], effects of stream restorations are poorly documented because of extremely limited post-restoration monitoring[16][4][6]). It is estimated that only 10% of stream restorations are monitored or assessed after implementation[1]. In an analysis of 345 stream restoration studies[4] found that conclusions on the effectiveness of restoration could not be drawn because of limited data on physical, chemical, and biological criteria collected primarily over short post-restoration time periods. For example, studies suggest that in-stream habitat modification can improve salmonid fish habitat and abundance, although the lack of statistically rigorous design and high variability in responses with different in-stream restoration structures and different species make broad conclusions about efficacy difficult[4]. It is not possible for many restoration studies to include monitoring in their projects due to funding limitations[14], but it is suggested those that can should adopt standard monitoring methods incorporating both stream structure and function responses, with measurements collected over long periods of time and across a wide range of environmental conditions (e.g., flood and drought years)[7][1][17][18]). Furthermore, measured environmental responses should be compared to multiple reference sites (minimally impacted systems of similar stream order and in the same ecoregion as the restored site) in order to fully assess restoration efficacy[7].
Stream Restoration Techniques
By their very nature, streams and rivers are dynamic ecosystems; thus, restoration efforts should focus on strategies designed to integrate dynamic physicochemical and biological processes[3][7][19][17][6]). For example, restoration objectives focused on re-establishing a dynamic stream ecosystem may include restoring natural sediment dynamics and water flow, restoring natural riparian plant communities, and restoring natural channel configurations[3].
When selecting a target site for restoration, it is suggested that watershed assessments be conducted to determine appropriate locations. In practice, however, target sites are most often selected because land is available for restoration[14]. It is important to note that if one restoration technique is conducted in multiple locations, the response of a system to restoration may not be the same because of site- and region-specific differences in geology, land use, climate, and biogeographic history[7][17]. For example, an analysis of 24 restoration projects found that stream restorations were more successful in improving stream macroinvertebrate communities when the restorations were conducted closer to intact forests, which provided a source of invertebrates to recolonize restored sites[20].
Bank stabilization |
Channel modification |
Fish reintroduction |
Flow augmentation |
Organic matter addition |
Revegetation of banks and riparian zones |
Land use regulation |
Soil stabilization |
Water quality improvements |
Water temperature alteration |
There are many types of stream restoration techniques that have been applied, and the type of restoration(s) can depend on the size and extent of the stressor, predominant land use in the watershed, position of the stream within the watershed, and the goals and objectives of the stakeholders[3][1][7]. Stream restoration goals and techniques are too numerous to discuss succinctly here (see Table 1). As one example, the five most common restoration techniques to improve habitat for salmonid fishes include habitat reconnection, road improvement, riparian management, in-stream habitat improvement, and nutrient enrichment[8].
One common restoration technique is the addition of coarse woody debris (CWD) to stream channels. CWD additions are a low-cost method to improve in-stream habitat[1]. In practice, nearby riparian trees are felled, cut, and staked in place within the stream channel. CWD additions can exert several positive effects on stream ecosystems including altering morphology and hydraulics of stream channels, creating habitat for benthic macroinvertebrates and fish, providing colonization surfaces for algae and other microorganisms, and increasing retention of sediment and organic matter, the latter of which is an important basal resource for many stream organisms[21][22][23][24][25]. Therefore, CWD additions have been used to improve in-stream habitat for aquatic organisms, primarily fish[8], and also, have been identified as a strategy to improve in-stream nitrogen retention[24][5]. However, a lack of comprehensive monitoring data has limited the ability to thoroughly evaluate the efficacy of this technique[19]. For example, Roni et al. (2002)[8] found that 41% of in-stream habitat improvement studies in the Pacific Northwest increased juvenile salmonid abundance; however, only about 20% of these studies quantified responses for >5 y, and responses varied across the restoration techniques examined[8].
Case Study: Assessment of In-Stream Restoration on DoD Land at Fort Benning Military Installation
At Fort Benning Military Installation (FBMI), military training activities (i.e., dismounted infantry tactics, tracked vehicle maneuvers) on sandy upland soils have led to erosion and high sedimentation rates in streams[26][11][27][28][2]. Specifically, streams at FBMI with higher watershed disturbance (defined as the % of the watershed with bare ground on slopes >5%) were found to have impaired water quality and reduced ecosystem function[27][29][24]. Therefore, an experimental restoration pilot project (i.e., CWD additions) was implemented in 2003 at FBMI to reduce the effects of military activities on stream ecosystem processes.
Both the short-term and long-term effects of CWD addition on stream ecosystem structure and function were evaluated in multiple highly disturbed streams at FBMI. The first project compared stream ecosystem processes before (2001-2003) and after (2003-2006) addition of CWD dams to stream channels. The second project examined responses 14 years after CWD additions (2017-2019) to determine whether there were any long-term benefits of CWD additions on in-stream stream structure and function.
CWD Additions at FBMI
CWD additions were installed in four headwater streams within Ft. Benning’s boundaries as part of a previously funded SERDP project[2]. Riparian trees (blackgum [Nyssa sylvatica] or white oak [Quercus alba]) were used for the CWD additions. Trees were felled on-site in August 2003, cut to the desired length, and then left on land to dry for 2-3 months prior to installation in the stream. In October 2003, cut trees (~10-20 cm in diameter, 1-2 m in length) were added in a “Z” configuration along a 100-150 m section within each stream (Figure 1). Three cut tree sections were used to make the “Z” design, with 10 to 15 of these “Z”-shaped woody debris dams installed along each stream length (~10 m apart), anchored in place using rebar stakes driven into the stream bed. The “Z” configuration was used to increase retention of organic matter and slow water flow to promote a stable stream bed. CWD additions approximately doubled the amount of in-stream CWD in restored streams[2].
One issue that was encountered several months after the CWD additions was that excessive sedimentation from storm events led to the burial of much of the added CWD in two of the four restored streams (Figure 2). Sedimentation and burial were likely exacerbated by high precipitation and streamflow in the post-restoration period, as the summer precipitation amounts in 2003, 2004 and 2005 were among the highest on record at that time, and streamflow was 5-8 times higher in the post-restoration period than the pre-restoration period[2]. Because of the CWD burial, the original CWD additions were augmented with a second addition of wood in 2004, such that the CWD coverage in these two streams was then triple the pre-restoration amount[2]. Burial of CWD dams was assessed in 2005 and 30% to 75% of the debris dams were buried in the restored streams.
Assessment of Restoration Efficacy
The first SERDP project used a before-after control-intervention (BACI) approach[30] to assess the effects of CWD addition on stream ecosystem structure and function. From July 2001 through October 2003, pre-restoration measurements were made at 8 stream sites on a monthly and seasonal basis. In October 2003, CWD dams were installed at 4 of the 8 stream sites, leaving 4 stream sites to serve as unrestored controls. Study sites were typical of low-gradient, sandy-bottomed streams of the southeastern Coastal Plain, and were all located within a ~10 km radius. Preliminary assessment of channel stability at several sites indicated a roughly similar degree of absolute bed movement (~2-6 mm) over the 2003 winter stormflow period[11]. From October 2003 through November 2006, post-restoration measurements were made at all 8 stream sites on a monthly and seasonal basis, enabling pre-restoration and post-restoration periods to be compared using the BACI method. Pre-restoration and post-restoration measurements included stream hydrodynamic properties (flashiness), water quality, nutrient (ammonium) uptake rates, whole-stream metabolism rates (i.e., gross primary production and ecosystem respiration), stream habitat condition (i.e., CWD coverage, benthic particulate organic matter [BPOM] standing stocks, streambed height dynamics), periphyton characteristics (i.e., chlorophyll a, ash-free dry mass, diatom taxa), and benthic macroinvertebrate community structure indices (i.e., Ephemeroptera, Plecoptera, Trichoptera [EPT] taxa, functional feeding groups, total density, total biomass, Florida Biotic Index).
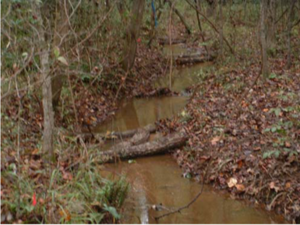
Beginning in May 2017, 14 years after the CWD additions, monthly and seasonal measurements resumed at 7 of the 8 stream sites (one unrestored site was not included in the reassessment because of high watershed disturbance after the initial project) to assess the long-term efficacy of CWD additions as a stream restoration technique. A subset of ecosystem structure and function measurements were collected from May 2017 through January 2019 and included measures of water quality, ammonium uptake rate, whole-stream metabolism, BPOM standing stocks, and macroinvertebrate richness and community structure.
Initial Effects of Restoration (2001-2006)
In general, there were no short-term changes to water quality parameters (i.e., pH, specific conductivity, and total suspended sediment, dissolved organic carbon, ammonium, nitrate, and phosphate concentrations) in restored streams immediately after restoration[2]. However, ammonium uptake rate, a measure of the rate at which nutrients cycle in streams, increased in restored streams within 1 month after restoration[24]. Ecosystem respiration also increased in restored streams for the first two years after CWD additions (2004-2005). Both the ammonium uptake and ecosystem respiration responses were likely due to increases in transient storage zone size, increased area for microbial colonization, and increased organic matter retention[24]. Gross primary production rates increased in 3 of the 4 restored streams from autumn 2004 through spring 2005, likely due to increased algal growth on CWD. However, by the end of the 3-year monitoring period, both GPP and ER rates in restored streams decreased to pre-restoration levels likely due to burial of the CWD[2]. Measurements of changes in streambed height over time revealed high among-stream variation and no overall effect of CWD additions on streambed stability[2].
Benthic macroinvertebrates are a diverse group of organisms, and several macroinvertebrate metrics are used to assess the health of stream ecosystems (Figure 3[31] Barbour et al. 1999, [32]. Macroinvertebrate assemblage responses to CWD additions were variable, with the effects varying by season and invertebrate metric (e.g., diversity, EPT taxa). For example, EPT density, which is often used as an indicator of stream health, increased in restored streams post-restoration, but only in winter[2]. There were no clear effects of restoration on total macroinvertebrate density. For additional information on the initial effects of restoration, see Mulholland et al. (2007)[2].
Long-Term Effects of Restoration (2017-2019)
From May 2017 through January 2019, post-restoration measurements were made ~14 years after the CWD additions in 7 streams (3 unrestored, 4 restored) on a monthly and seasonal basis (compare Figure 4 to Figure 5). This study design enabled the long-term assessment of CWD dam additions as a restoration tool on stream ecosystem structure and function. The most immediate finding is that the CWD dams are still in place 14 y after their initial installation; however, preliminary analyses suggest no long-term benefits of CWD dams on stream ecosystem structure and function.
References
- ^ 1.0 1.1 1.2 1.3 1.4 1.5 1.6 Bernhardt, E.S., Palmer, M.A., Allan, J.D., Alexander, G., Barnas, K., Brooks, S., Carr, J., Clayton, S., Dahm, C., Follstad-Shah, J. and Galat, D., 2005. Synthesizing US river restoration efforts. Science. Vol. 308, Issue 5722, pp. 636-637. doi: 10.1126/science.1109769
- ^ 2.00 2.01 2.02 2.03 2.04 2.05 2.06 2.07 2.08 2.09 2.10 2.11 Mulholland, P.J., Feminella, J.W., Lockaby, B.G. and Hollon, G.L., 2007. Riparian Ecosystem Management at Military Installations: Determination of Impacts and Evaluation of Restoration and Enhancement Strategies. Final Technical Report SI-1186. Pp.161. Report.pdf
- ^ 3.00 3.01 3.02 3.03 3.04 3.05 3.06 3.07 3.08 3.09 3.10 3.11 National Research Council, 1992. Restoration of Aquatic Ecosystems: Science, Technology, and Public Policy. National Academies Press. doi: 10.17226/1807
- ^ 4.0 4.1 4.2 4.3 4.4 Roni, P., Hanson, K. and Beechie, T., 2008. Global review of the physical and biological effectiveness of stream habitat rehabilitation techniques. North American Journal of Fisheries Management, 28(3), pp.856-890. doi: 10.1577/M06-169.1
- ^ 5.0 5.1 5.2 Craig, L.S., Palmer, M.A., Richardson, D.C., Filoso, S., Bernhardt, E.S., Bledsoe, B.P., Doyle, M.W., Groffman, P.M., Hassett, B.A., Kaushal, S.S. and Mayer, P.M., 2008. Stream restoration strategies for reducing river nitrogen loads. Frontiers in Ecology and the Environment, 6(10), pp.529-538. doi: 10.1890/070080
- ^ 6.0 6.1 6.2 6.3 6.4 Bernhardt, E.S. and Palmer, M.A., 2011. River restoration: the fuzzy logic of repairing reaches to reverse catchment scale degradation. Ecological applications, 21(6), pp.1926-1931. doi: 10.1890/10-1574.1
- ^ 7.0 7.1 7.2 7.3 7.4 7.5 7.6 7.7 Palmer, M.A., Bernhardt, E.S., Allan, J.D., Lake, P.S., Alexander, G., Brooks, S., Carr, J., Clayton, S., Dahm, C.N., Shah, J.F. and Galat, D.L., 2005. Standards for ecologically successful river restoration. Journal of applied ecology, 42(2), pp.208-217. doi: 10.1111/j.1365-2664.2005.01004.x
- ^ 8.0 8.1 8.2 8.3 8.4 Roni, P., Beechie, T.J., Bilby, R.E., Leonetti, F.E., Pollock, M.M. and Pess, G.R., 2002. A review of stream restoration techniques and a hierarchical strategy for prioritizing restoration in Pacific Northwest watersheds. North American Journal of Fisheries Management, 22(1), pp.1-20. <0001:AROSRT>2.0.CO;2 doi: 10.1577/1548-8675(2002)022<0001:AROSRT>2.0.CO;2
- ^ US Environmental Protection Agency (USEPA), 2006. Wadeable Streams Assessment: A Collaborative Survey of the Nation's Streams. EPA. Office of Research and Development, Office of Water, Washington DC 20460. EPA 841-B-06-002. Report.pdf
- ^ Allan, J.D., 2004. Landscapes and riverscapes: the influence of land use on stream ecosystems. Annu. Rev. Ecol. Evol. Syst., 35, pp.257-284. doi: 10.1146/annurev.ecolsys.35.120202.110122
- ^ 11.0 11.1 11.2 Maloney, K.O., Mulholland, P.J. and Feminella, J.W., 2005. Influence of catchment-scale military land use on stream physical and organic matter variables in small southeastern plains catchments (USA). Environmental Management, 35(5), pp.677-691. doi: 10.1007/s00267-005-8651-5
- ^ Walsh, C.J., Roy, A.H., Feminella, J.W., Cottingham, P.D., Groffman, P.M. and Morgan, R.P., 2005. The urban stream syndrome: current knowledge and the search for a cure. Journal of the North American Benthological Society, 24(3), pp.706-723. doi: 10.1899/04-028.1
- ^ Rabalais, N.N., Turner, R.E. and Wiseman Jr, W.J., 2002. Gulf of Mexico hypoxia, aka “The dead zone”. Annual Review of ecology and Systematics, 33(1), pp.235-263. doi: 10.1146/annurev.ecolsys.33.010802.150513
- ^ 14.0 14.1 14.2 Sudduth, E.B., Meyer, J.L. and Bernhardt, E.S., 2007. Stream restoration practices in the southeastern United States. Restoration Ecology, 15(3), pp.573-583. doi: 10.1111/j.1526-100X.2007.00252.x
- ^ Stanford, J.A., Ward, J.V., Liss, W.J., Frissell, C.A., Williams, R.N., Lichatowich, J.A. and Coutant, C.C., 1996. A general protocol for restoration of regulated rivers. Regulated Rivers: Research & Management, 12(4‐5), pp.391-413. <391::AID-RRR436>3.0.CO;2-4 doi: 10.1002/(SICI)1099-1646(199607)12:4/5<391::AID-RRR436>3.0.CO;2-4
- ^ Bilby, R.E. and Ward, J.W., 1991. Characteristics and function of large woody debris in streams draining old-growth, clear-cut, and second-growth forests in southwestern Washington. Canadian Journal of Fisheries and Aquatic Sciences, 48(12), pp.2499-2508. doi: 10.1139/f91-291
- ^ 17.0 17.1 17.2 Wohl, E., Angermeier, P.L., Bledsoe, B., Kondolf, G.M., MacDonnell, L., Merritt, D.M., Palmer, M.A., Poff, N.L. and Tarboton, D., 2005. River restoration. Water Resources Research, 41(10). doi: 10.1029/2005WR003985
- ^ Lake, P.S., Bond, N. and Reich, P., 2007. Linking ecological theory with stream restoration. Freshwater Biology, 52(4), pp.597-615. doi: 10.1111/j.1365-2427.2006.01709.x
- ^ 19.0 19.1 Thompson, D.M., 2005. The history of the use and effectiveness of instream structures in the United States. Humans as Geologic Agents, 16, p.35. doi: /10.1130/2005.4016(04)
- ^ Sundermann, A., Stoll, S. and Haase, P., 2011. River restoration success depends on the species pool of the immediate surroundings. Ecological Applications, 21(6), pp.1962-1971. doi: 10.1890/10-0607.1
- ^ Bilby, R.E. and Likens, G.E., 1980. Importance of organic debris dams in the structure and function of stream ecosystems. Ecology, 61(5), pp.1107-1113. doi: 10.2307/1936830
- ^ Smock, L.A., Metzler, G.M. and Gladden, J.E., 1989. Role of debris dams in the structure and functioning of low‐gradient headwater streams. Ecology, 70(3), pp.764-775. doi: 10.2307/1940226
- ^ Bilby, R.E. and Ward, J.W., 1991. Characteristics and function of large woody debris in streams draining old-growth, clear-cut, and second-growth forests in southwestern Washington. Canadian Journal of Fisheries and Aquatic Sciences, 48(12), pp.2499-2508. doi: 10.1139/f91-291
- ^ 24.0 24.1 24.2 24.3 24.4 Roberts, B.J., Mulholland, P.J. and Houser, J.N., 2007. Effects of upland disturbance and instream restoration on hydrodynamics and ammonium uptake in headwater streams. Journal of the North American Benthological Society, 26(1), pp.38-53. [38:EOUDAI2.0.CO;2 doi:10.1899/0887-3593(2007)26[38:EOUDAI]2.0.CO;2]
- ^ Roni, P., Beechie, T., Pess, G. and Hanson, K., 2014. Wood placement in river restoration: fact, fiction, and future direction. Canadian Journal of Fisheries and Aquatic Sciences, 72(3), pp.466-478. doi: 10.1139/cjfas-2014-0344
- ^ Lockaby, B.G., Governo, R., Schilling, E., Cavalcanti, G. and Hartsfield, C., 2005. Effects of sedimentation on soil nutrient dynamics in riparian forests. Journal of Environmental Quality, 34(1), pp.390-396. doi:10.2134/jeq2005.0390
- ^ 27.0 27.1 Houser, J.N., Mulholland, P.J. and Maloney, K.O., 2005. Catchment disturbance and stream metabolism: patterns in ecosystem respiration and gross primary production along a gradient of upland soil and vegetation disturbance. Journal of the North American Benthological Society, 24(3), pp.538-552. doi: 10.1899/04-034.1
- ^ Houser, J.N., Mulholland, P.J. and Maloney, K.O., 2006. Upland disturbance affects headwater stream nutrients and suspended sediments during baseflow and stormflow. Journal of Environmental Quality, 35(1), pp.352-365. doi:10.2134/jeq2005.0102
- ^ Mulholland, P.J., Houser, J.N. and Maloney, K.O., 2005. Stream diurnal dissolved oxygen profiles as indicators of in-stream metabolism and disturbance effects: Fort Benning as a case study. Ecological Indicators, 5(3), pp.243-252. doi: 10.1016/j.ecolind.2005.03.004
- ^ Stewart-Oaten, A., Murdoch, W.W. and Parker, K.R., 1986. Environmental impact assessment:" Pseudoreplication" in time?. Ecology, 67(4), pp.929-940. doi: 10.2307/1939815
- ^ Barbour, M.T., Gerritsen, J., Snyder, B.D. and Stribling, J.B., 1999. Rapid bioassessment protocols for use in streams and wadeable rivers: periphyton, benthic macroinvertebrates and fish (Vol. 339). Washington, DC: US Environmental Protection Agency, Office of Water. EPA 841-B-99-02. Report.pdf
- ^ Maloney, K.O. and Feminella, J.W., 2006. Evaluation of single-and multi-metric benthic macroinvertebrate indicators of catchment disturbance over time at the Fort Benning Military Installation, Georgia, USA. Ecological Indicators, 6(3), pp.469-484. doi: 10.1016/j.ecolind.2005.06.003